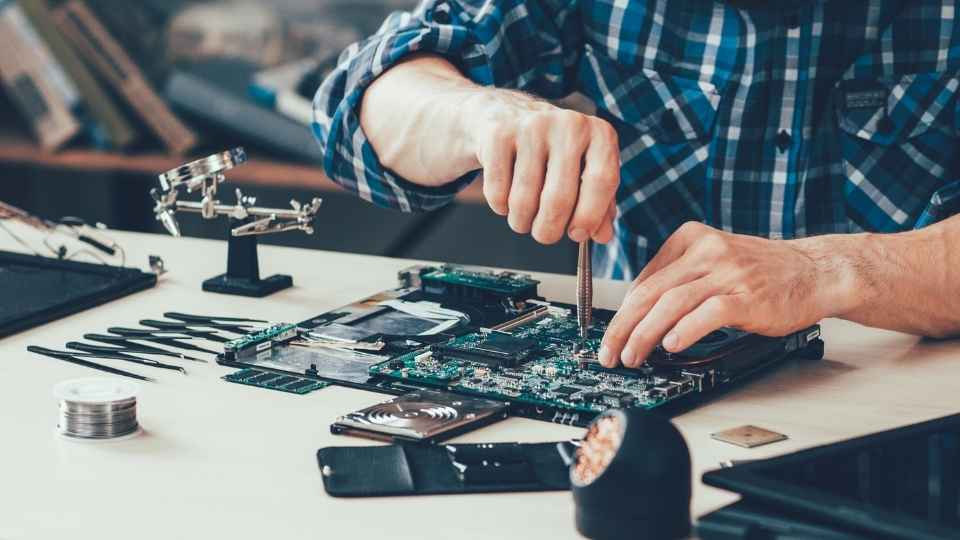
As an electrical engineer fascinated by the wonders of semiconductors, I often find myself pondering the age-old question: silicon or germanium? These two elements have long been at the heart of modern electronics, powering everything from smartphones to computers.
In this article, we will delve into the intricacies of semiconductors and explore why silicon has become the dominant choice. However, we will also venture into the realm of germanium and discuss its potential as a viable alternative.
So sit back, relax, and prepare to uncover the magic behind these remarkable materials.
Key Takeaways
- Silicon is the most commonly used semiconductor material due to its abundance, excellent electrical properties, and relatively low cost.
- Germanium, although widely used in early transistor technology, has been largely replaced by silicon.
- The P-N junction, formed by merging P-type and N-type semiconductor materials, allows for precise control over conductivity characteristics.
- Silicon's abundant availability, thermal conductivity, and well-established manufacturing processes make it a reliable choice for semiconductor devices.
The Basics of Semiconductors
If you want to understand the basics of semiconductors, you'll need to learn about silicon and germanium.
These two elements are at the heart of modern electronics, allowing us to create devices that have revolutionized our lives.
Silicon is the most commonly used semiconductor material due to its abundance and excellent electrical properties. It has a crystalline structure that allows it to conduct electricity under certain conditions but also act as an insulator when necessary.
Germanium, on the other hand, was widely used in early transistor technology before silicon took over. It has similar properties to silicon but is less abundant and more expensive.
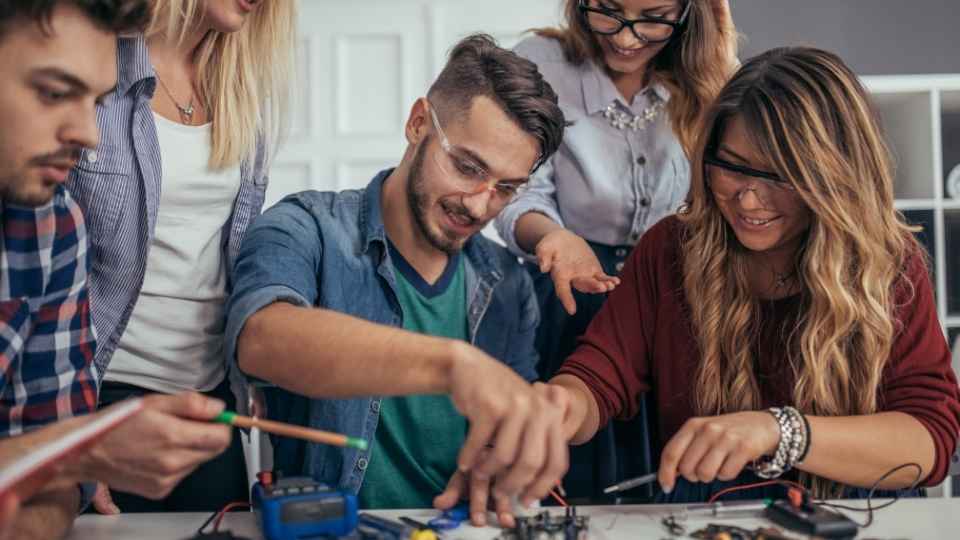
Both materials play a crucial role in the development of electronic devices, giving us the freedom to explore new possibilities and push boundaries in technology.
Understanding the P-N Junction
The P-N junction is a key element in understanding how semiconductors work. It plays a crucial role in the functionality of devices like diodes and transistors. This junction is formed by merging two different types of semiconductor materials: P-type and N-type.
In the P-type region, there's an excess of positively charged holes, while in the N-type region, there's an abundance of negatively charged electrons.
When these two regions are brought together, something remarkable happens. Electrons from the N-type material diffuse into the P-type material, resulting in a charge imbalance at the junction. This creates a depletion region where no current can flow unless an external voltage is applied.
By controlling the voltage across this P-N junction, we can manipulate its conductivity characteristics. A positive voltage applied to the P-side will attract electrons from the N-side, allowing current to flow through the device. Conversely, a negative voltage repels electrons and inhibits current flow.
Understanding this fundamental concept empowers us to design and build electronic devices with incredible precision and versatility. Whether it's powering your smartphone or enabling renewable energy systems, it's all thanks to our understanding of P-N junctions in semiconductors.
The Role of Silicon in Semiconductors
As a semiconductor material, silicon plays a crucial role in the functioning of electronic devices. Its abundant availability and relatively low cost make it an attractive choice for manufacturing semiconductors on a large scale.
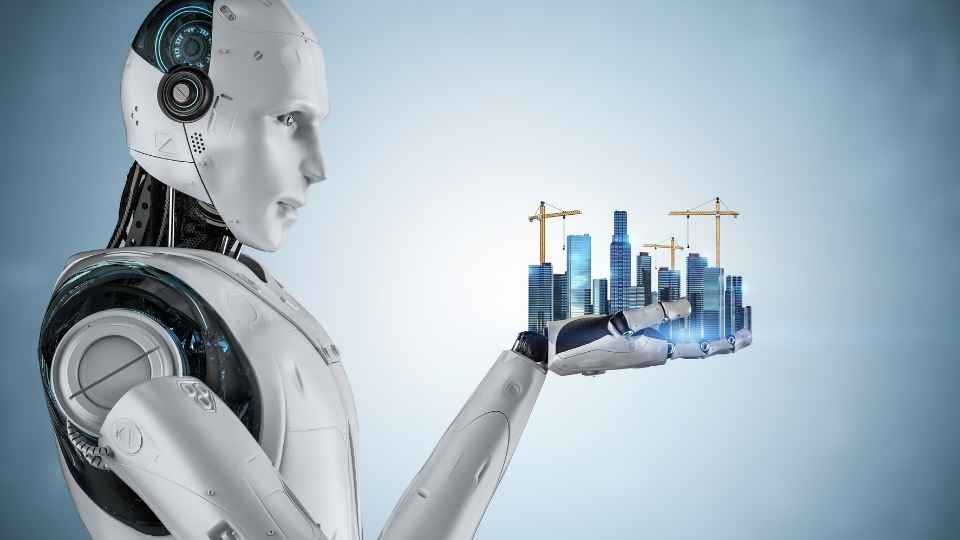
Furthermore, silicon exhibits high thermal conductivity, allowing it to efficiently dissipate heat generated during device operation.
Lastly, its superior electrical properties enable precise control over the flow of current, making it an ideal material for designing transistors and other essential components of modern electronics.
Silicon's Abundant Availability
Silicon's abundant availability makes it a popular choice for semiconductors. Its widespread presence in the Earth's crust, combined with its excellent electrical properties, has made silicon the cornerstone of modern technology. Here are four reasons why silicon's abundance is crucial for our freedom-driven society:
Cost-effectiveness: Silicon is readily available and relatively inexpensive to extract and refine, making it economically viable for mass production of semiconductor devices.
Scalability: The abundance of silicon allows for large-scale manufacturing of semiconductors, enabling the production of countless electronic components that power our everyday devices.
Versatility: Silicon can be doped with impurities to modify its electrical properties, making it suitable for a wide range of applications in transistors, diodes, and integrated circuits.
Reliability: Due to its abundance and well-established manufacturing processes, silicon-based semiconductors have been extensively tested and proven reliable over decades.
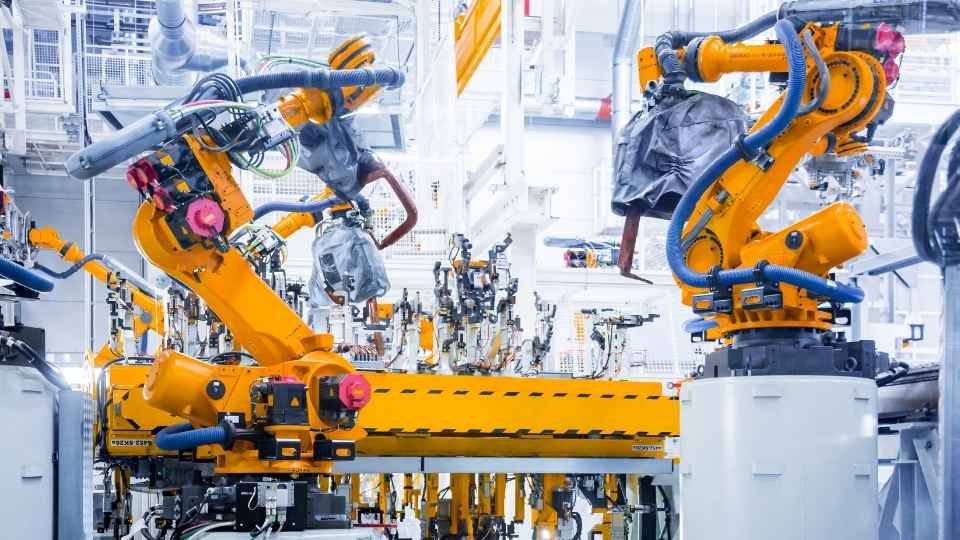
Silicon's High Thermal Conductivity
Silicon's high thermal conductivity allows for efficient heat dissipation in electronic devices. This property is crucial as excessive heat can degrade the performance and reliability of electronic components. Silicon, a group IV semiconductor, possesses one of the highest thermal conductivities among all materials. With a value of approximately 150 W/m·K, it surpasses most metals commonly used in electronics. The unique crystal structure of silicon enables efficient transfer of heat by facilitating the movement of phonons, which are atomic vibrations responsible for thermal energy transmission. As a result, silicon-based devices can withstand higher power densities without overheating or experiencing performance degradation. This exceptional thermal conductivity ensures that our electronic devices remain cool and reliable even under demanding conditions.
Transitioning into the subsequent section about silicon's superior electrical properties, this remarkable material also exhibits excellent electrical characteristics that make it an ideal choice for semiconductor applications.
Silicon's Superior Electrical Properties
As we delve deeper into the world of semiconductors, it becomes evident that silicon possesses remarkable electrical properties. Here are four reasons why silicon's electrical properties make it a superior choice:
High carrier mobility: Silicon exhibits excellent electron and hole mobility, allowing for efficient charge transport within the material.
Wide energy bandgap: With a bandgap of 1.1 eV, silicon can easily switch between conductive and insulating states, enabling precise control over electronic devices.
Low leakage current: Silicon's low intrinsic carrier concentration results in minimal leakage current, reducing power consumption and enhancing device performance.
Stable oxide layer formation: Silicon readily forms a stable oxide layer (silicon dioxide) on its surface, providing protection against environmental factors and ensuring long-term device reliability.
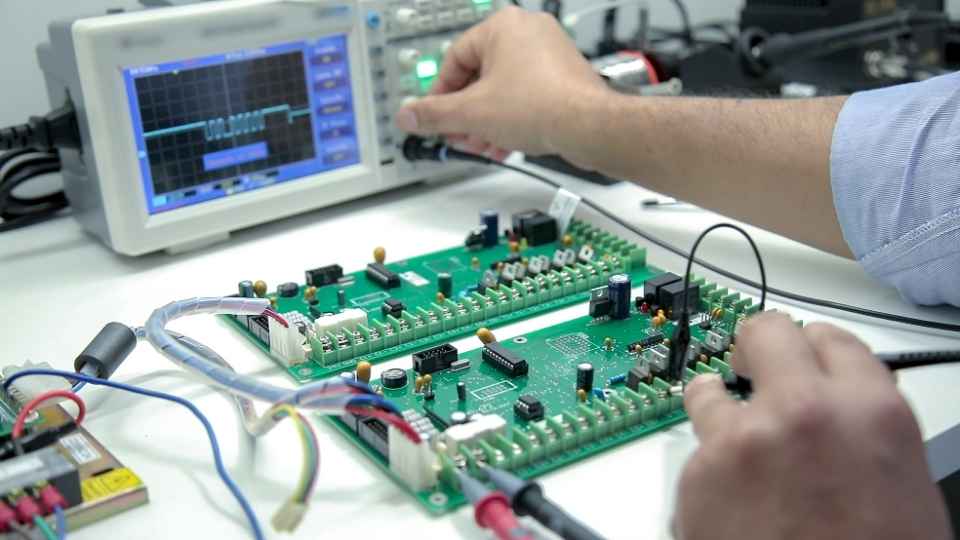
These exceptional electrical properties of silicon have revolutionized electronics and paved the way for numerous technological advancements. However, as we explore alternative materials, such as germanium, we can evaluate whether there are better options available to meet our evolving needs.
Exploring Germanium as an Alternative
Germanium, once considered a promising alternative to silicon, has several unique properties. It's a chemical element with atomic number 32, located in Group 14 of the periodic table. Germanium's crystalline structure is similar to that of silicon, making it suitable for use in semiconductors.
However, its key advantage lies in its higher electron mobility compared to silicon. This means that electrons can move through germanium more easily, resulting in faster electronic devices. Germanium also has a narrower bandgap than silicon, allowing it to absorb and emit light more efficiently. Additionally, germanium exhibits less resistance at high temperatures than silicon does.
These properties make germanium an attractive option for applications such as infrared detectors and optical communication systems.
Now let's compare the properties of silicon and germanium to determine which one is more suitable for various semiconductor applications.
Comparing Silicon and Germanium Properties
Now let's take a closer look at how the properties of silicon and germanium compare to determine which one would be more suitable for various semiconductor applications.
Bandgap: Silicon has a larger bandgap (1.12 eV) compared to germanium (0.67 eV), making it better suited for high-temperature applications where thermal energy can excite electrons across the bandgap.
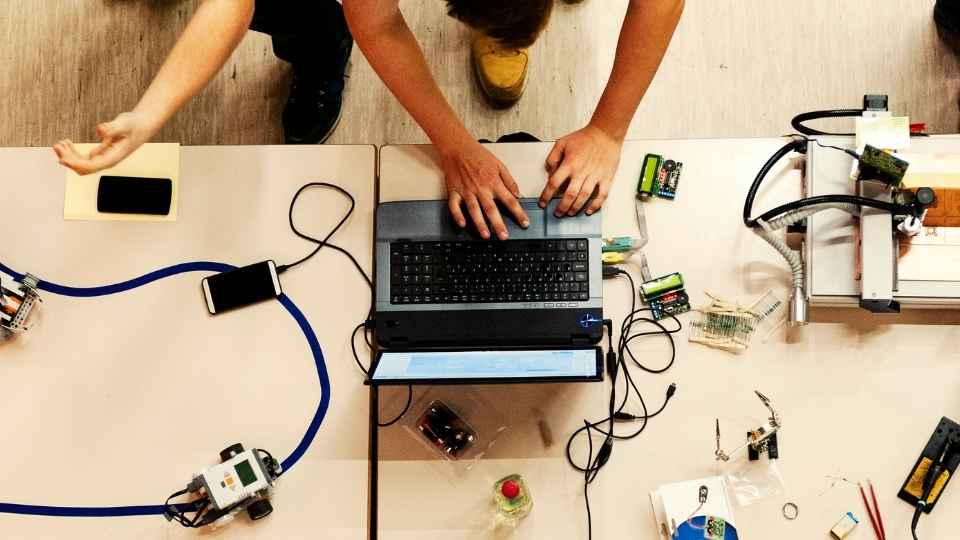
Mobility: Germanium exhibits higher electron and hole mobility compared to silicon, allowing for faster charge carrier movement and hence potentially better performance in certain high-frequency applications.
Thermal conductivity: Silicon has nearly three times higher thermal conductivity than germanium, making it more efficient in dissipating heat generated during device operation.
Availability and cost: Silicon is abundantly available and relatively inexpensive compared to germanium, making it the preferred choice for most commercial semiconductor applications.
Based on these factors, while germanium may have some advantages in specific situations, silicon remains the go-to material due to its superior overall performance, lower cost, and wide availability in the market.
Applications of Silicon and Germanium in Electronics
As an engineer, I'm fascinated by the myriad applications of silicon and germanium in electronics. These two semiconductor materials possess unique electrical properties that make them essential for various electronic devices.
Additionally, their compatibility with semiconductor devices allows for efficient integration into complex circuits.
Furthermore, the differences in thermal conductivity between silicon and germanium play a crucial role in managing heat dissipation within electronic systems.
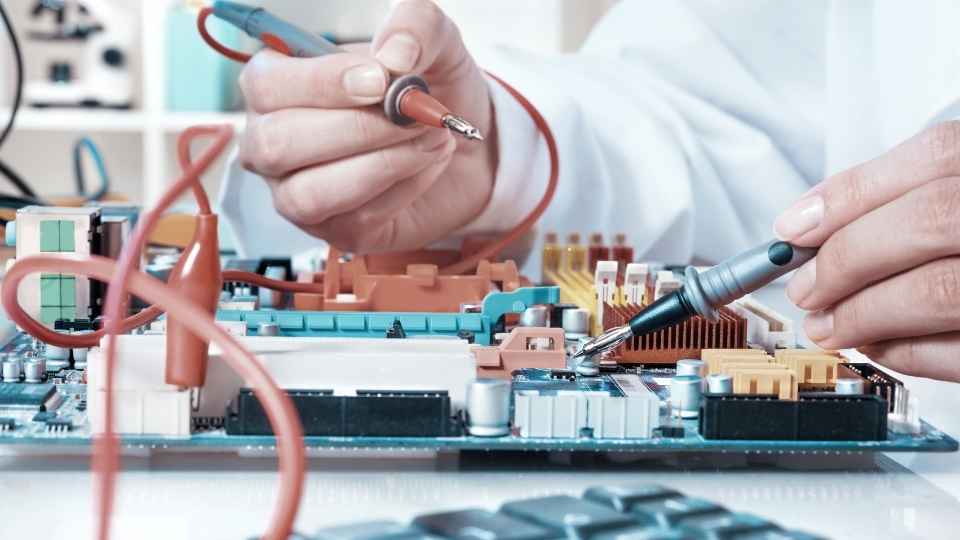
Unique Electrical Properties
The unique electrical properties of semiconductors make them essential components in modern technology. Semiconductors have the ability to conduct electricity under certain conditions, making them versatile materials for various applications. Here are four key properties that make semiconductors so valuable:
Bandgap: Semiconductors have a specific energy gap between their valence and conduction bands. This allows them to switch between conducting and insulating states, enabling the creation of electronic devices like transistors.
Doping: By introducing impurities into the crystal lattice of a semiconductor, its electrical conductivity can be modified. Doping with elements like boron or phosphorus allows control over the number of charge carriers, crucial for designing electronic circuits.
Thermoelectricity: Semiconductors exhibit the Seebeck effect, converting temperature differences into electric voltage. This property is harnessed in thermoelectric generators to transform waste heat into useful electricity.
Photovoltaics: When light strikes a semiconductor material, it generates electron-hole pairs that create an electric current flow. Photovoltaic cells capitalize on this phenomenon to convert sunlight into electrical energy.
Understanding these unique properties empowers us to unlock new technological advancements and harness the freedom they bring in our daily lives.
Semiconductor Device Compatibility
Understanding the compatibility of semiconductor devices is crucial for designing efficient electronic circuits. When integrating different types of semiconductors into a circuit, it's important to consider their electrical characteristics, such as voltage and current requirements. Failure to do so can result in inefficiencies or even damage to the circuit components. Additionally, factors like temperature stability and power dissipation must be taken into account when selecting compatible devices.
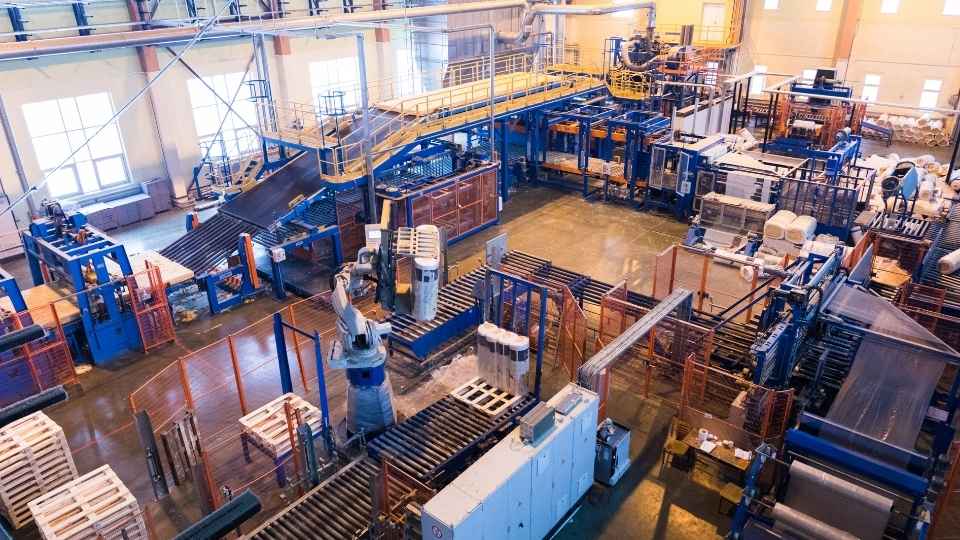
One aspect that needs careful attention is the thermal conductivity differences between various semiconductors. Thermal conductivity determines how efficiently heat can be dissipated from a device, preventing overheating and potential failure. Silicon generally has higher thermal conductivity compared to germanium due to its crystal structure. This means that silicon-based devices have better heat dissipation capabilities, making them suitable for high-power applications where thermal management is critical.
Considering these compatibility factors ensures that electronic circuits function optimally while maintaining reliability and longevity.
Thermal Conductivity Differences
To optimize the efficiency of your electronic circuits, it's crucial to consider the differences in thermal conductivity between various semiconductor materials.
Here are four key points to keep in mind:
Silicon: Silicon is the most commonly used semiconductor material due to its high thermal conductivity. It can efficiently dissipate heat and ensure reliable performance even under demanding conditions.
Germanium: Although not as popular as silicon, germanium has a higher intrinsic carrier concentration and lower bandgap energy. However, it has lower thermal conductivity compared to silicon, which may lead to increased heat accumulation and potential device failure.
Thermal Interface Materials: Using suitable thermal interface materials can significantly enhance heat transfer between semiconductors and heatsinks or other cooling components. These materials fill gaps and improve contact, minimizing thermal resistance.
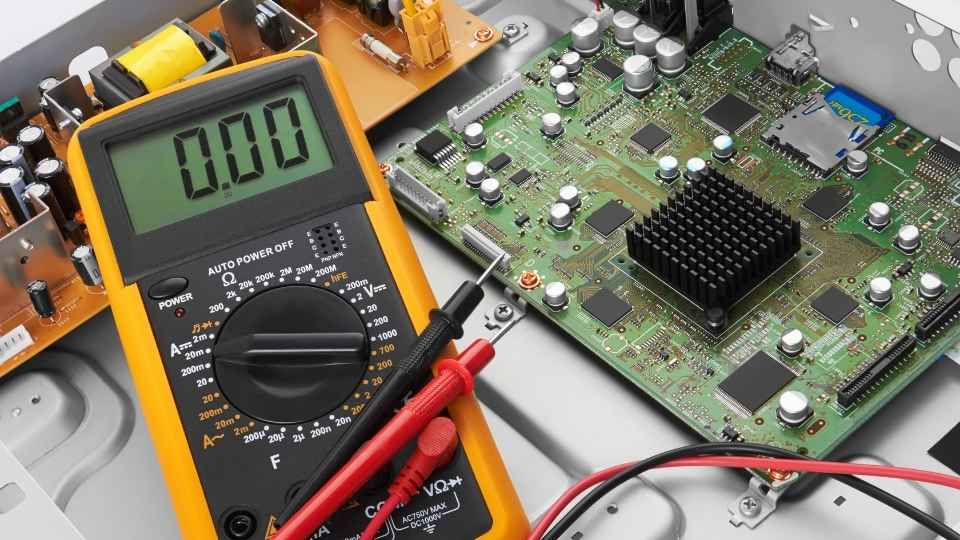
Heat Dissipation Techniques: Employing effective heat dissipation techniques such as adding heatsinks or using active cooling methods like fans or liquid cooling can further improve the overall performance and reliability of your electronic circuits.
Considering these factors will help you make informed decisions when selecting semiconductor materials for your applications while ensuring optimal circuit efficiency and longevity.
Frequently Asked Questions
How Do Semiconductors Work?
Semiconductors work by manipulating the flow of electrons through their material structure. By doping them with impurities, we can control their conductivity and create electronic devices like transistors, which form the backbone of modern technology.
What Are the Different Types of Semiconductors?
There are different types of semiconductors, each with unique properties. These include silicon, germanium, gallium arsenide, and more. Understanding the characteristics of each helps us determine their suitability for various electronic applications.
What Are Some Common Applications of Semiconductors?
Some common applications of semiconductors include computer chips, solar cells, LED lights, and transistors. These devices utilize the unique properties of semiconductors to enable functions such as data processing, energy conversion, and signal amplification.
How Are Silicon and Germanium Different in Terms of Their Properties?
In terms of their properties, silicon and germanium differ in various ways. Silicon has a higher melting point, wider bandgap, and better thermal conductivity compared to germanium. These differences impact their suitability for different semiconductor applications.
Why Is Silicon More Commonly Used in Electronic Devices Compared to Germanium?
Silicon is more commonly used in electronic devices compared to germanium due to its availability, stability at higher temperatures, and superior electrical properties such as higher carrier mobility and lower leakage current.
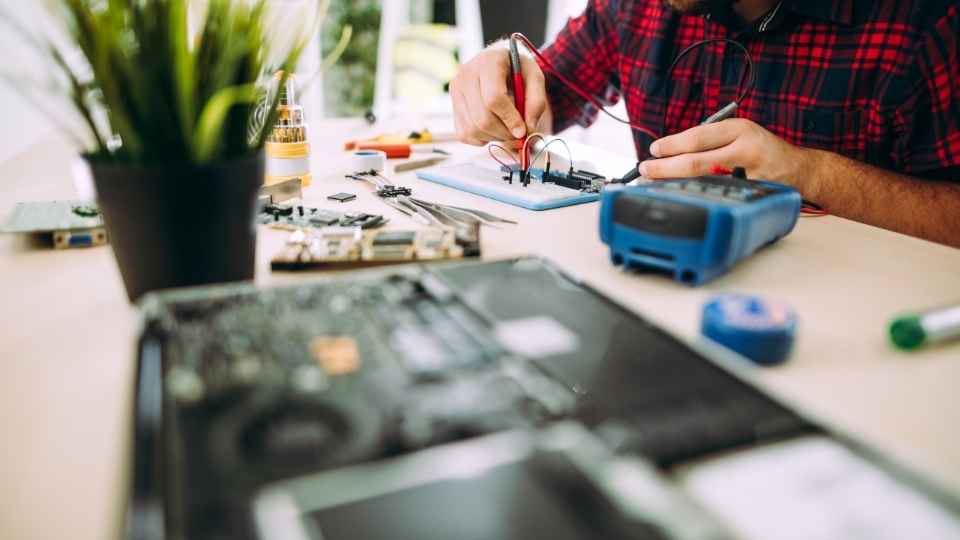